Quantum Computing: Breaking the Barriers of Technology
Welcome to the fascinating world of quantum computing, where the impossible becomes possible and technology takes a giant leap forward! Imagine a realm where computers can solve complex problems in seconds, a feat that would take classical computers eons to achieve. This article delves into the revolutionary field of quantum computing, exploring its fundamental principles, potential applications, and the myriad challenges it faces in transforming technology as we know it. Whether you are a tech enthusiast or a curious learner, get ready to embark on an exciting journey into the quantum realm!
To grasp the essence of quantum computing, we must first dive into the mysterious world of quantum mechanics. At its core, quantum mechanics is a branch of physics that deals with the behavior of matter and energy at the smallest scales—think atoms and subatomic particles. Here are some key principles that form the backbone of quantum computing:
- Superposition: Unlike classical bits, which can be either 0 or 1, qubits can exist in multiple states at once. This means they can represent both 0 and 1 simultaneously, dramatically increasing computational power.
- Entanglement: This phenomenon allows qubits that are entangled to be interdependent, no matter the distance between them. Changing the state of one qubit instantly influences its entangled partner, enabling faster information processing.
- Quantum Bits (Qubits): The fundamental units of quantum information, qubits are what set quantum computers apart from their classical counterparts.
So, what makes up a quantum computer? Understanding its key components is essential to appreciate its capabilities. A quantum computer consists of several essential elements:
- Qubits: The building blocks of quantum computing.
- Quantum Gates: These are the operations that manipulate qubits, similar to how classical logic gates operate on bits.
- Quantum Circuits: A series of quantum gates that work together to perform complex computations.
Let’s take a closer look at qubits. These remarkable entities are the heart of quantum computing. Unlike classical bits, which can only be in one of two states, qubits can exist in a state of superposition. This unique property allows quantum computers to perform multiple calculations simultaneously, exponentially increasing their processing power. Imagine trying to find your way through a maze; a classical computer would have to try each path one by one, while a quantum computer could explore all paths at once!
There are several types of qubits, each with its advantages and challenges:
Type of Qubit | Advantages | Challenges |
---|---|---|
Superconducting Qubits | Fast operation and scalability | Susceptible to noise |
Trapped Ions | High fidelity and long coherence times | Complex control systems |
Topological Qubits | Inherent error resistance | Still largely theoretical |
Now, let’s talk about quantum gates. These are the operations that manipulate qubits, akin to how classical logic gates manipulate bits. Quantum gates perform functions such as flipping the state of a qubit or entangling two qubits together. By combining these gates in various configurations, quantum computers can execute complex algorithms that would be impossible for classical systems.
Quantum algorithms are where the real magic happens! Algorithms like Shor’s algorithm and Grover’s algorithm showcase the potential of quantum computing:
- Shor's Algorithm: This algorithm can factor large numbers exponentially faster than the best-known classical algorithms, posing a significant threat to current encryption methods.
- Grover's Algorithm: This algorithm allows for faster searching of unsorted databases, making it invaluable for optimization problems.
These algorithms hold promise for various fields, including cryptography, optimization, and drug discovery, potentially revolutionizing how we approach complex problems.
Despite its potential, quantum computing faces significant challenges. Issues like error rates, decoherence, and the need for advanced quantum error correction techniques threaten its reliability. Without addressing these challenges, the dream of practical quantum computing may remain just that—a dream.
To ensure quantum computations are stable and reliable, researchers are developing various quantum error correction methods. These techniques aim to detect and correct errors that occur during quantum operations, ensuring that the results remain accurate.
Another major hurdle is the scalability of quantum computers. As we attempt to build larger and more powerful quantum systems, we encounter hardware limitations and the need for robust infrastructure. Achieving practical scalability is crucial for the widespread adoption of quantum technologies.
The future of quantum computing is filled with promise and speculation. As researchers continue to make breakthroughs, we can anticipate significant advancements that could change the landscape of technology forever. From commercialization to integration into various industries, the impact of quantum computing will be profound.
Different sectors are already preparing for quantum computing technology. Industries such as finance, healthcare, and logistics are exploring how quantum computing can optimize their operations and improve decision-making processes.
However, with great power comes great responsibility. The societal implications of quantum computing are vast, raising ethical considerations about privacy, security, and the potential to reshape industries and job markets. As we stand on the brink of this technological revolution, it’s vital to navigate these challenges thoughtfully.
Q: What is quantum computing?
A: Quantum computing is a type of computing that utilizes the principles of quantum mechanics to process information in ways that classical computers cannot.
Q: How does quantum computing differ from classical computing?
A: Quantum computing uses qubits that can exist in multiple states simultaneously, while classical computing relies on bits that are either 0 or 1.
Q: What are some applications of quantum computing?
A: Quantum computing has potential applications in cryptography, optimization, drug discovery, and complex simulations, among others.
Q: What are the main challenges facing quantum computing?
A: Major challenges include error rates, decoherence, and scalability issues that need to be addressed for practical use.
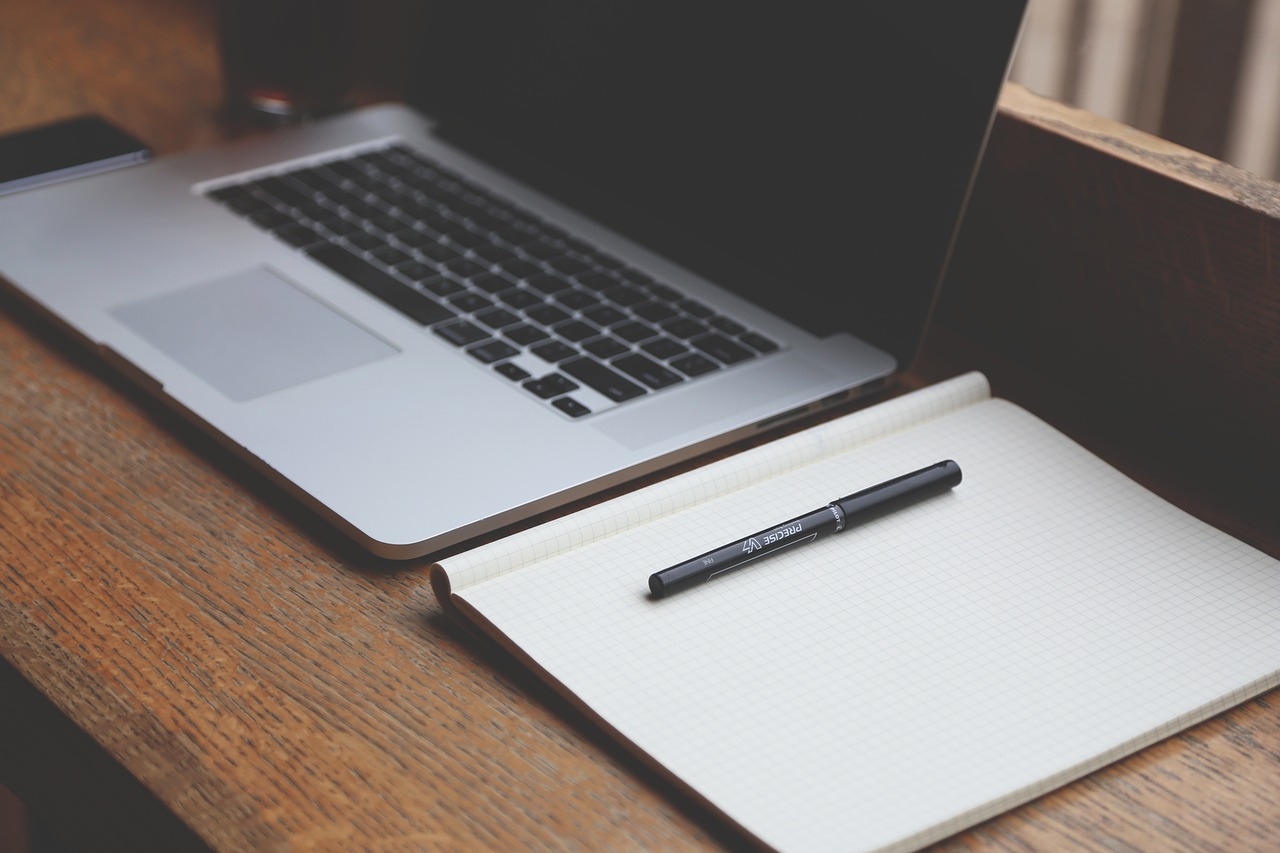
Understanding Quantum Mechanics
Quantum mechanics is like stepping into a world where the rules of reality bend and twist in fascinating ways. Imagine a place where a particle can exist in multiple states at once or where two particles can be interconnected regardless of the distance separating them. These mind-boggling concepts form the bedrock of quantum computing, a field that promises to revolutionize technology as we know it. At its core, quantum mechanics introduces us to the concepts of superposition and entanglement, which are crucial for understanding how quantum computers operate.
Let's break it down. Superposition is the idea that a quantum bit, or qubit, can be in a state of 0, 1, or both 0 and 1 simultaneously. This is akin to flipping a coin and having it land in a state where it shows both heads and tails at the same time. In classical computing, a bit can only be one or the other, but qubits can harness this duality to perform complex calculations at lightning speed. This property enables quantum computers to process vast amounts of information much more efficiently than traditional computers.
Then there’s entanglement, which Einstein famously referred to as “spooky action at a distance.” When qubits become entangled, the state of one qubit instantly influences the state of another, no matter how far apart they are. This interconnectedness allows quantum computers to solve problems in ways that classical computers simply cannot. For instance, if you have two entangled qubits, measuring one will give you immediate information about the other, even if it’s light-years away. This kind of relationship is not just fascinating; it’s a game-changer for computational speed and efficiency.
To illustrate these principles, consider a simple analogy: think of a traditional computer as a very efficient librarian who can only read one book at a time. In contrast, a quantum computer is like a magical librarian who can read multiple books simultaneously and instantly share information across all the books. This ability to multitask exponentially increases the potential for solving complex problems.
The role of quantum bits (qubits) cannot be overstated. Unlike classical bits, which are limited to binary states, qubits can exist in a continuum of states due to their quantum nature. This unique characteristic is what allows quantum computers to tackle problems that would take classical computers millennia to solve. As we delve deeper into the world of quantum computing, we’ll explore how these principles are applied in real-world scenarios and the implications they hold for the future of technology.
In summary, understanding quantum mechanics is essential for grasping the potential of quantum computing. The concepts of superposition and entanglement not only challenge our perceptions of reality but also open up a realm of possibilities for technological advancement. As we continue this journey, we will uncover the key components of quantum computers and how they differ from their classical counterparts.
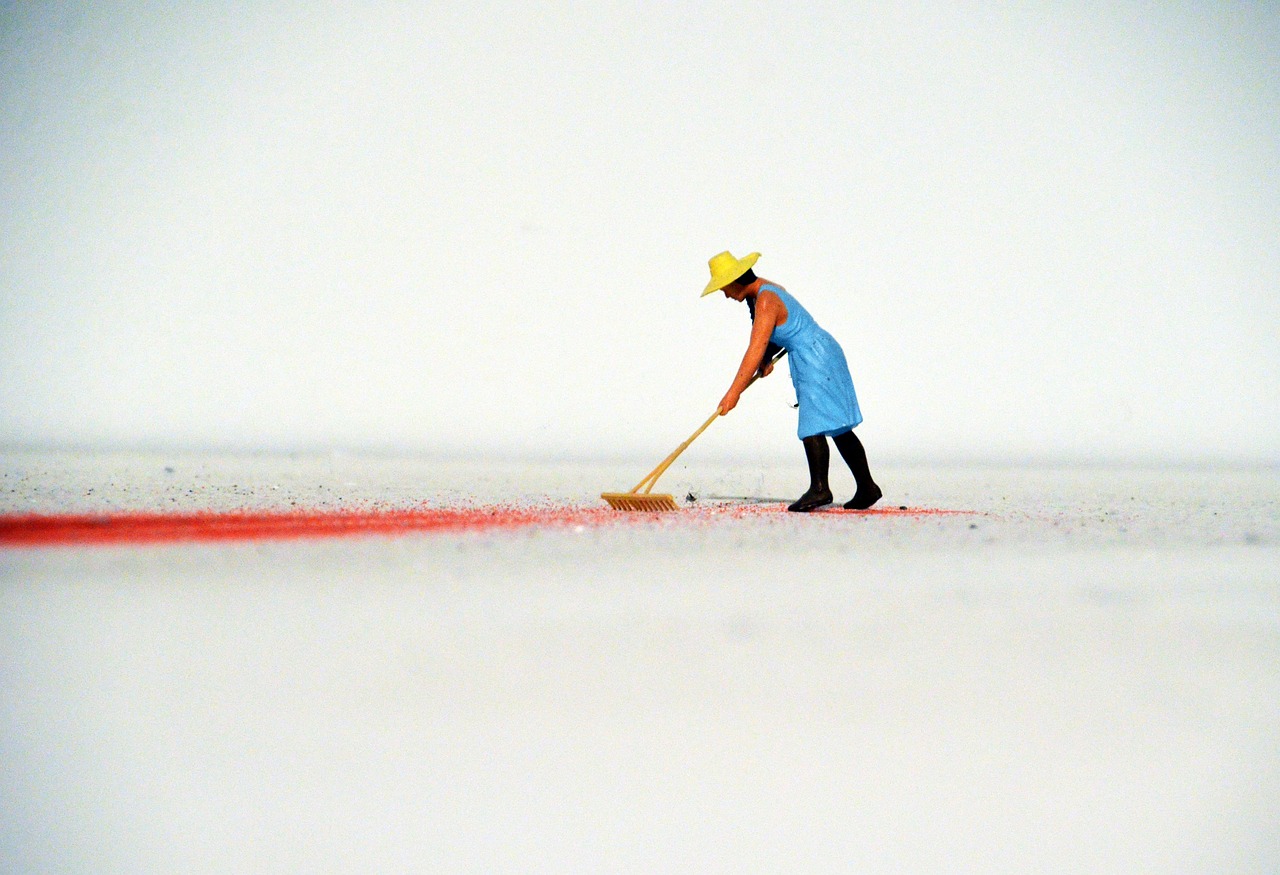
Key Components of Quantum Computers
Quantum computers are not just an upgraded version of classical computers; they are a completely different breed. To truly appreciate their power, we need to dive into the key components that make them tick. At the heart of quantum computing lies the qubit, which is the fundamental unit of quantum information. Unlike classical bits that can either be 0 or 1, qubits can exist in a state of superposition, meaning they can be both 0 and 1 at the same time. This unique property allows quantum computers to process vast amounts of data simultaneously, leading to unparalleled computational speed.
In addition to qubits, quantum computers utilize quantum gates to manipulate the state of qubits. These gates are the quantum equivalent of classical logic gates and perform operations that change the probabilities associated with qubit states. The arrangement of these gates forms quantum circuits, which are essential for executing quantum algorithms. The design and implementation of these circuits are crucial, as they determine how effectively a quantum computer can solve complex problems.
To illustrate the differences between classical and quantum components, consider the following table:
Component | Classical Computing | Quantum Computing |
---|---|---|
Basic Unit | Bit | Qubit |
State | 0 or 1 | 0, 1, or both (superposition) |
Operations | Logic Gates | Quantum Gates |
Data Processing | Sequential | Parallel |
Another critical aspect of quantum computers is their reliance on entanglement. When qubits become entangled, the state of one qubit is directly related to the state of another, no matter how far apart they are. This phenomenon allows for faster communication and more complex calculations, as changes to one qubit can instantaneously affect its entangled partner. It’s as if you have a pair of magic dice; when you roll one, the other instantly shows the same number, defying the conventional understanding of distance and time.
As we explore the key components of quantum computers, it becomes clear that the synergy between qubits, quantum gates, and entanglement is what sets quantum computing apart from its classical counterpart. The ability to harness these properties opens up a world of possibilities, from revolutionizing cryptography to optimizing complex logistical problems. However, with great power comes great responsibility, and the intricacies of these components also introduce challenges that researchers are actively working to overcome.
- What is a qubit?
A qubit is the basic unit of quantum information, capable of representing both 0 and 1 simultaneously due to superposition. - How do quantum gates work?
Quantum gates manipulate the states of qubits, allowing them to perform complex operations necessary for quantum algorithms. - What is entanglement?
Entanglement is a quantum phenomenon where the state of one qubit is linked to another, regardless of the distance separating them. - Why is quantum computing faster?
Quantum computing can perform many calculations at once due to superposition and entanglement, making it exponentially faster than classical computing for certain tasks.
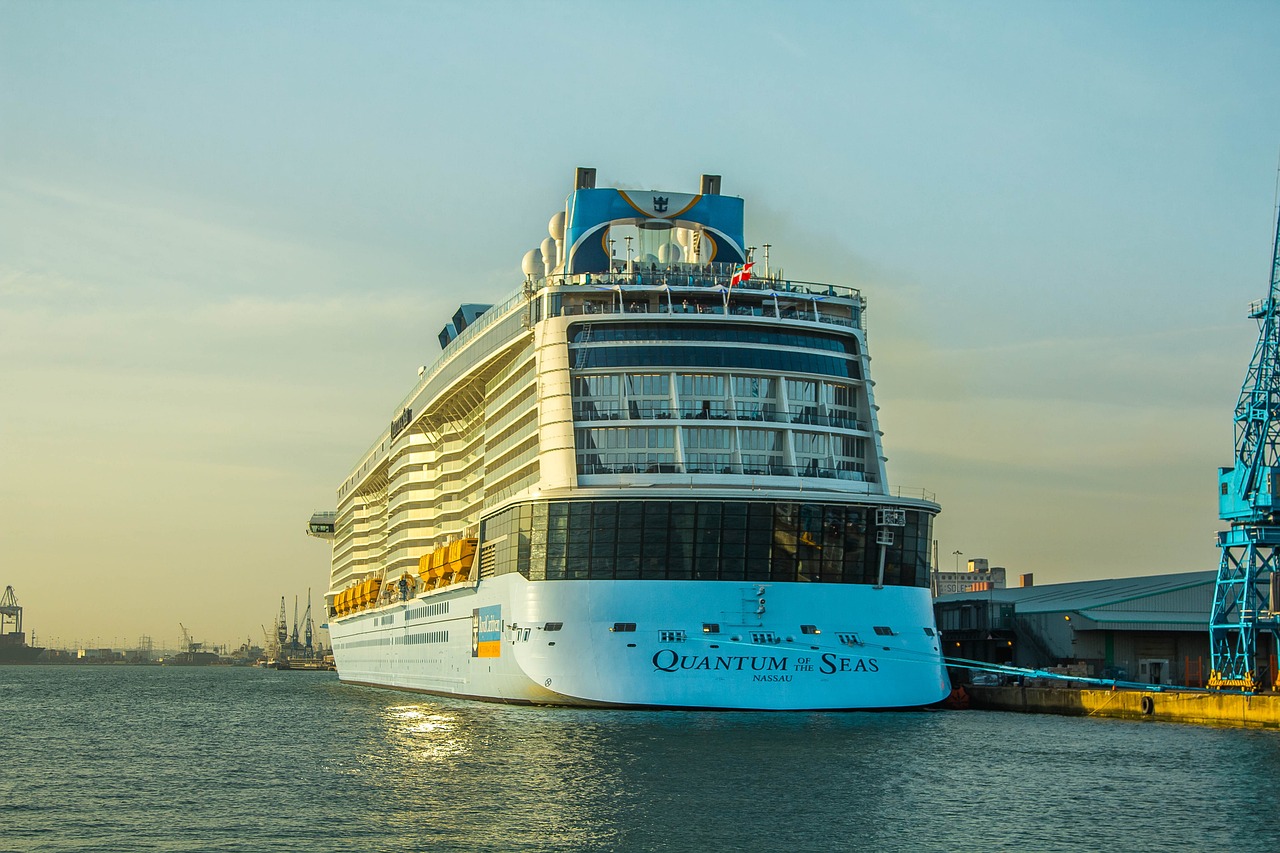
Qubits: The Building Blocks
When we dive into the fascinating world of quantum computing, we quickly realize that the heart of this revolutionary technology lies in qubits. Unlike classical bits, which can only represent a 0 or a 1, qubits are capable of existing in multiple states simultaneously, thanks to the principle of superposition. This unique property allows quantum computers to process vast amounts of information at speeds that classical computers can only dream of. Imagine trying to find a needle in a haystack; while a classical computer would sift through each piece of hay one by one, a quantum computer can explore numerous possibilities at once, making it exponentially faster.
But what exactly are qubits made of? They can be realized using various physical systems, each with its own advantages and challenges. Some common types of qubits include:
- Superconducting Qubits: These are made from materials that exhibit superconductivity at very low temperatures. They are currently among the most widely used in quantum computers.
- Trapped Ion Qubits: Here, ions are trapped using electromagnetic fields and manipulated with lasers. This type offers high fidelity but can be challenging to scale.
- Topological Qubits: These are still largely theoretical but promise greater stability and error resistance, making them an exciting area of research.
Each type of qubit has its own set of challenges. For instance, while superconducting qubits are relatively easy to fabricate, they are prone to noise and interference from their environment. On the other hand, trapped ions offer excellent coherence times but can be cumbersome to manage as the number of qubits increases. This brings us to the next critical aspect of qubits: their entanglement. When qubits become entangled, the state of one qubit becomes dependent on the state of another, no matter how far apart they are. This phenomenon not only enhances the computational power of quantum systems but also opens the door to new types of quantum algorithms.
As we explore the building blocks of quantum computing, it’s essential to understand how qubits interact through quantum gates. These gates are the operations that manipulate qubits, allowing them to perform complex calculations. Just as classical logic gates form the backbone of traditional computing, quantum gates are crucial for executing quantum algorithms. They enable qubits to switch states, create entanglement, and perform a variety of operations that classical bits simply cannot.
In conclusion, qubits are the fundamental units of quantum information, and their unique properties set the stage for the extraordinary capabilities of quantum computers. As researchers continue to explore and refine these building blocks, we inch closer to unlocking the full potential of quantum computing, paving the way for breakthroughs that could revolutionize fields ranging from cryptography to drug discovery.
1. What is a qubit?
A qubit, or quantum bit, is the basic unit of quantum information that can exist in multiple states simultaneously, unlike a classical bit, which can only be a 0 or a 1.
2. How do qubits work?
Qubits leverage quantum mechanical phenomena such as superposition and entanglement to perform calculations at speeds far beyond those of classical computers.
3. What are the different types of qubits?
Common types of qubits include superconducting qubits, trapped ion qubits, and topological qubits, each with unique properties and challenges.
4. Why are qubits important in quantum computing?
Qubits are essential because they enable quantum computers to perform complex calculations and solve problems that are currently infeasible for classical computers.
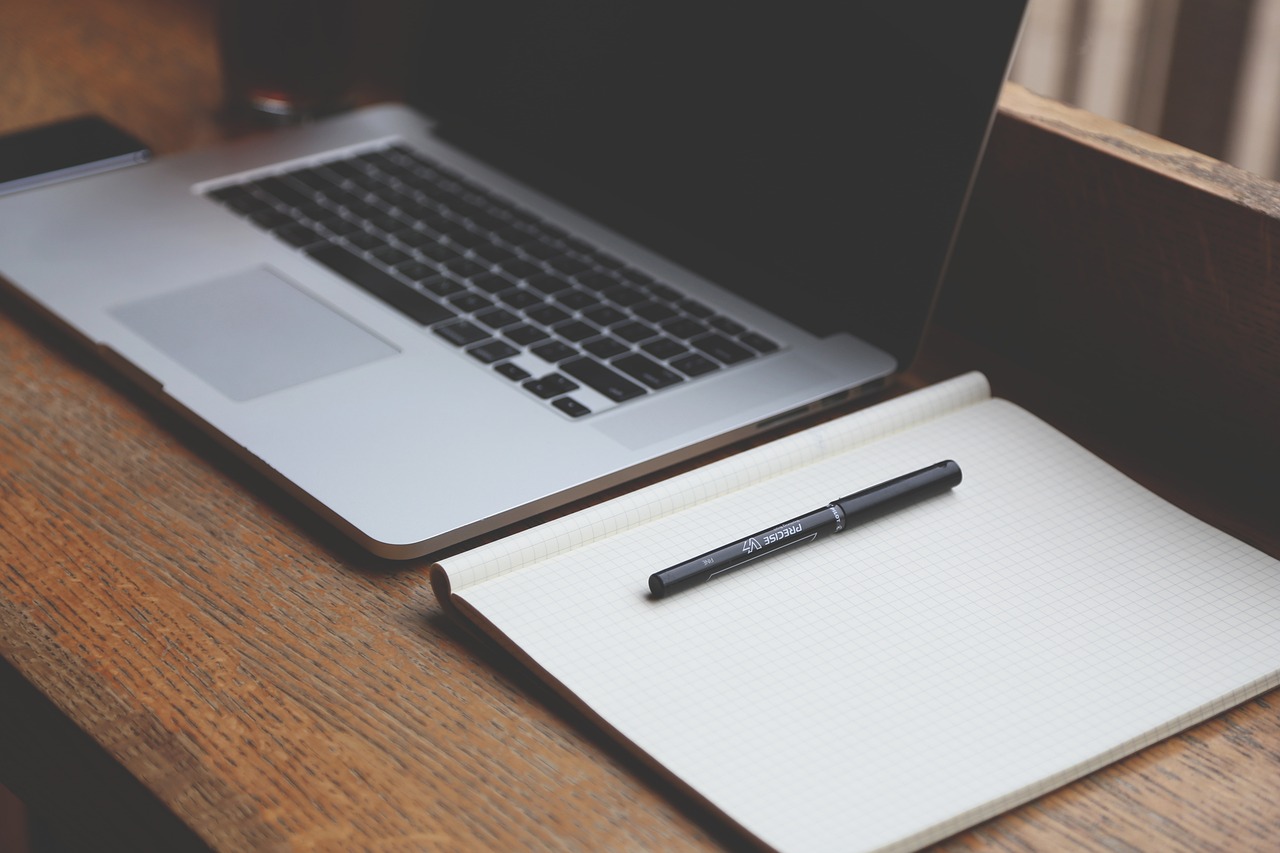
Types of Qubits
When diving into the fascinating realm of quantum computing, one cannot overlook the pivotal role of qubits. These are not just your run-of-the-mill bits that we encounter in classical computing; qubits possess unique properties that allow them to exist in multiple states simultaneously, thanks to the principles of quantum mechanics. But did you know that there are various types of qubits, each with its own set of advantages and challenges? Understanding these types is crucial for grasping how quantum computers operate and their potential impact on technology.
One of the most prevalent types of qubits is the superconducting qubit. These qubits are made from materials that exhibit superconductivity at very low temperatures. They are created using tiny circuits that can oscillate between two energy states, which allows them to represent both 0 and 1 at the same time. The beauty of superconducting qubits lies in their relatively fast operation speed and the ability to be integrated into existing semiconductor technology. However, they require extremely low temperatures to function, which poses significant challenges in terms of both cost and practicality.
Next up, we have trapped ion qubits. These qubits utilize ions that are confined in a small space using electromagnetic fields. By using lasers to manipulate the energy levels of these ions, researchers can perform quantum operations. One of the standout features of trapped ion qubits is their long coherence times, meaning they can retain their quantum state for a longer period, which is vital for complex computations. Nevertheless, the challenge lies in the scalability of this technology since controlling and maintaining a large number of trapped ions is a daunting task.
Then there are topological qubits, which represent a more theoretical approach to qubit design. These qubits are based on the principles of topology, a branch of mathematics that studies the properties of space that are preserved under continuous transformations. The key advantage of topological qubits is their inherent resistance to errors caused by environmental noise, which is a significant hurdle in quantum computing. However, the technology is still in its infancy, and practical implementations are yet to be realized.
To summarize, here are the three main types of qubits we discussed:
- Superconducting Qubits: Fast operation but require low temperatures.
- Trapped Ion Qubits: Long coherence times but challenging to scale.
- Topological Qubits: Error-resistant but still theoretical.
Each type of qubit brings something unique to the table, and the race is on to determine which will ultimately prove most effective for practical quantum computing applications. As researchers continue to explore these diverse qubit types, we can expect to see exciting advancements that will push the boundaries of technology even further. The journey of quantum computing is just beginning, and the types of qubits we choose to develop will play a crucial role in shaping its future.
- What is a qubit? A qubit is the basic unit of quantum information that can exist in multiple states simultaneously, unlike a classical bit.
- Why are different types of qubits important? Different qubit types offer various advantages and challenges, influencing the performance and scalability of quantum computers.
- What is the most common type of qubit used today? Superconducting qubits are currently among the most widely used in experimental quantum computing.
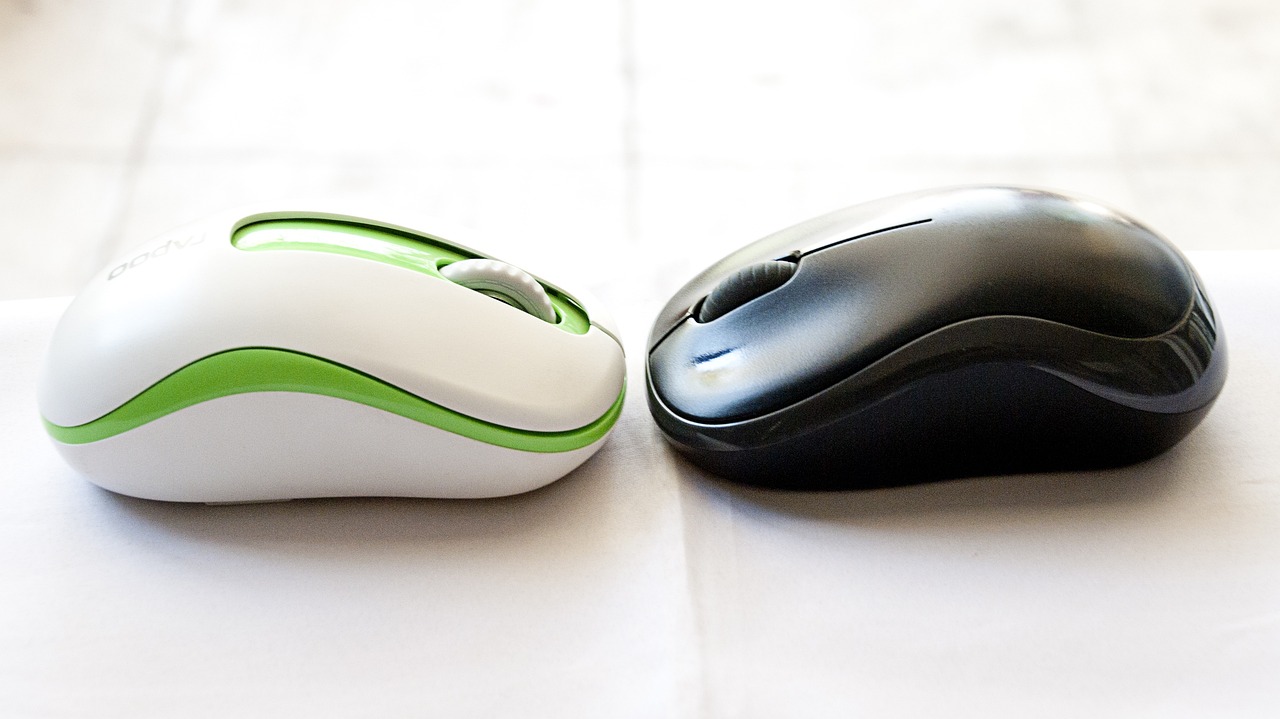
Quantum Gates Explained
Quantum gates are the fundamental building blocks of quantum circuits, much like how classical logic gates are the foundation of classical computing. They are responsible for manipulating quantum bits, or qubits, through various operations. Unlike classical gates, which operate on bits that are either 0 or 1, quantum gates can operate on qubits that exist in a state of superposition, allowing them to represent both 0 and 1 simultaneously. This unique property enables quantum computers to perform complex calculations at speeds unimaginable with classical computers.
To understand how quantum gates function, it's essential to grasp some basic concepts. Each quantum gate performs a specific operation that transforms the state of qubits, and these transformations can be visualized using matrices. For instance, a simple quantum gate called the Hadamard gate takes a qubit in the state |0⟩ and transforms it into a superposition of |0⟩ and |1⟩. This is crucial for quantum algorithms that require the exploration of multiple possibilities simultaneously.
There are several types of quantum gates, each serving different purposes. Some of the most common include:
- Pauli Gates: These gates, including X, Y, and Z, are analogous to classical NOT gates but operate on qubits in a more complex manner.
- Controlled Gates: These gates, such as the CNOT (Controlled NOT) gate, allow one qubit to control the operation of another, creating entanglement—a key feature of quantum computing.
- Phase Gates: These gates add a phase shift to the qubit's state, which is vital in various quantum algorithms.
Quantum gates are typically represented in a quantum circuit diagram, where qubits are depicted as horizontal lines and gates as boxes or symbols that act on these lines. The operation of the gates can be expressed mathematically using unitary matrices, which ensure that the transformation preserves the probability amplitude of the qubit states. This is crucial, as the fidelity of quantum computations relies on maintaining the integrity of these probabilities.
In practice, quantum gates are executed in sequences to form quantum algorithms. The interplay of these gates allows quantum computers to perform operations that would take classical computers an impractical amount of time. For example, Shor's algorithm, which can factor large numbers exponentially faster than the best-known classical algorithms, relies heavily on the precise manipulation of qubits through quantum gates.
As we continue to explore the potential of quantum computing, understanding quantum gates is essential. They are not just tools; they are the keys that unlock the vast potential of quantum algorithms, enabling us to solve problems that were once thought impossible. With advancements in quantum gate technology, we are on the brink of a new era in computing that could revolutionize industries and change the way we approach complex challenges.
Q1: What is a quantum gate?
A quantum gate is a basic building block of quantum circuits that performs operations on qubits, manipulating their states to enable quantum computations.
Q2: How do quantum gates differ from classical logic gates?
Unlike classical gates that operate on bits (0 or 1), quantum gates manipulate qubits that can exist in multiple states simultaneously due to superposition.
Q3: Why are quantum gates important?
Quantum gates are crucial for executing quantum algorithms, allowing quantum computers to solve complex problems much faster than classical computers.
Q4: Can quantum gates create entanglement?
Yes, certain quantum gates, like the CNOT gate, can create entangled states between qubits, which is a fundamental aspect of quantum computing.
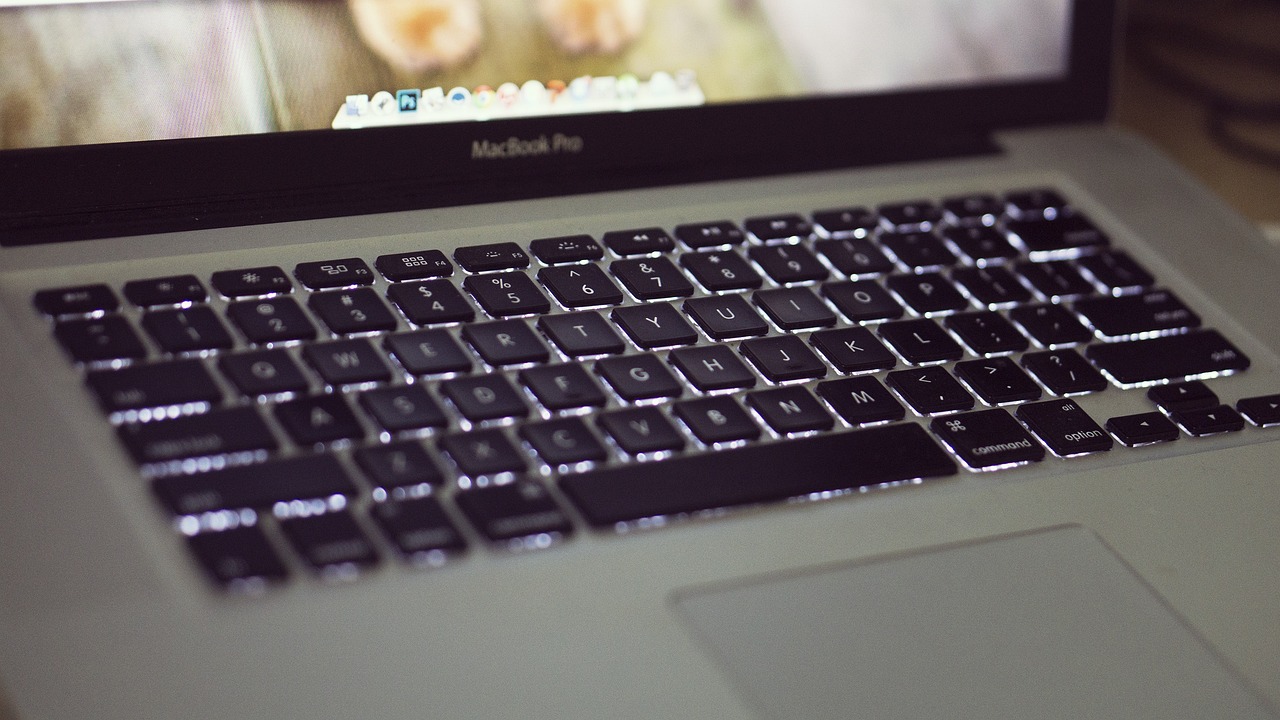
Quantum Algorithms and Their Applications
Quantum algorithms are at the heart of what makes quantum computing such an exciting field. Unlike classical algorithms, which operate on bits that can be either 0 or 1, quantum algorithms leverage the unique properties of qubits. This allows them to perform calculations at speeds that are simply unimaginable with traditional computers. For instance, algorithms like Shor's and Grover's have caught the attention of researchers and industries alike due to their potential to revolutionize fields such as cryptography, optimization, and drug discovery.
Shor's algorithm, developed by mathematician Peter Shor in 1994, is particularly noteworthy. It demonstrates how quantum computers can factor large integers exponentially faster than the best-known classical algorithms. This capability poses a significant threat to conventional cryptographic systems that rely on the difficulty of factoring large numbers to secure sensitive information. Imagine being able to crack codes that currently protect everything from online banking to national security—this is the kind of disruption that Shor's algorithm could bring.
On the other hand, Grover's algorithm offers a different kind of power. It provides a quadratic speedup for unstructured search problems. In simpler terms, if you have a database of N items, Grover's algorithm can find a specific item in approximately √N steps, compared to N steps in classical computing. This could have profound implications for fields like data analysis and artificial intelligence, where searching through vast datasets is a common task.
To illustrate the impact of these algorithms, let’s take a look at a few application areas:
Application Area | Quantum Algorithm | Potential Impact |
---|---|---|
Cryptography | Shor's Algorithm | Breaking RSA encryption, leading to the need for quantum-resistant algorithms. |
Search Problems | Grover's Algorithm | Faster data retrieval, enhancing AI and machine learning capabilities. |
Drug Discovery | Variational Quantum Eigensolver | Simulating molecular interactions to expedite drug development. |
The potential applications of quantum algorithms extend far beyond these examples. In finance, for instance, quantum computing could optimize trading strategies and risk assessments by processing vast amounts of data in real-time. In logistics, companies could find the most efficient routes for delivery trucks, saving time and fuel costs. Moreover, in the realm of artificial intelligence, quantum algorithms could lead to breakthroughs in machine learning by enabling more complex models to be trained faster.
However, while the promise of quantum algorithms is tantalizing, it’s important to remember that we are still in the early stages of this technology. Many of these algorithms are theoretical at this point, and practical implementations are still being developed. As researchers continue to explore the capabilities of quantum computing, we can expect to see more innovative algorithms emerge, each with the potential to unlock new possibilities across various industries.
- What is a quantum algorithm? A quantum algorithm is a step-by-step procedure for solving a problem using the principles of quantum mechanics, specifically designed to run on quantum computers.
- How do quantum algorithms differ from classical algorithms? Quantum algorithms can process information in ways that classical algorithms cannot, leveraging phenomena like superposition and entanglement to achieve faster computation.
- What are some real-world applications of quantum algorithms? Real-world applications include cryptography, drug discovery, optimization problems, and complex data analysis.
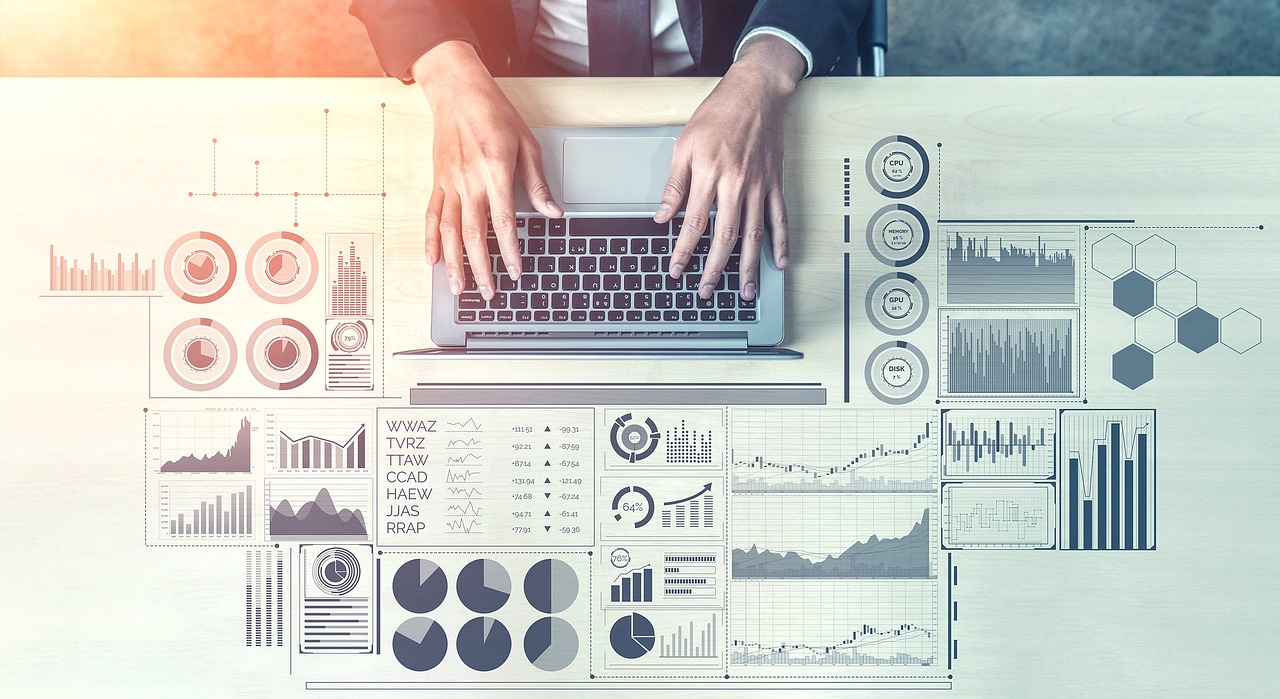
Challenges in Quantum Computing
Quantum computing, while a fascinating frontier in technology, is not without its **challenges**. As researchers and engineers delve deeper into the intricacies of quantum mechanics, they encounter a series of hurdles that must be overcome to realize the full potential of this revolutionary technology. One of the most significant obstacles is the **error rates** associated with quantum computations. Unlike classical computers, which can execute millions of calculations with a high degree of accuracy, quantum computers are susceptible to errors due to the fragile nature of qubits. These errors can arise from a variety of sources, including environmental noise and operational inaccuracies.
Another critical issue is **decoherence**, which refers to the loss of quantum coherence in qubits. In simpler terms, decoherence occurs when qubits lose their quantum state and revert to classical states, making it challenging to maintain the delicate balance needed for quantum computations. This phenomenon can happen in mere fractions of a second, which means that any calculations relying on qubits must be completed almost instantaneously. To put it in perspective, imagine trying to balance a spinning top while simultaneously performing complex calculations; if the top wobbles too much, it will fall over, and all your calculations will be lost.
To tackle these issues, researchers are developing **advanced quantum error correction techniques**. These methods aim to identify and rectify errors during computation, ensuring that the output remains reliable. For instance, one popular approach is the use of **redundancy**, where the same qubit state is encoded across multiple physical qubits. This way, if one qubit fails, the information can still be retrieved from the others, much like how a backup system works in classical computing.
Another challenge lies in the **scalability** of quantum computers. While small-scale quantum systems have shown promise, scaling these systems to a practical level poses significant hurdles. The current hardware limitations make it difficult to increase the number of qubits without introducing more errors or instability. Additionally, robust infrastructure is required to support these complex systems, including advanced cooling technologies and precise control mechanisms. Imagine trying to build a skyscraper on a shaky foundation; no matter how innovative your design is, if the base isn't solid, the entire structure is at risk of collapsing.
In summary, while quantum computing holds immense promise, it is essential to address these challenges head-on. From mitigating error rates and decoherence to developing scalable systems, the path to a fully functional quantum computer is fraught with difficulties. However, with ongoing research and innovation, the vision of harnessing quantum power for practical applications may one day become a reality.
- What is the primary challenge in quantum computing? The primary challenges include error rates, decoherence, and scalability issues.
- How does error correction work in quantum computing? Error correction involves encoding qubit states across multiple qubits to safeguard against errors.
- Why is decoherence a problem for quantum computers? Decoherence leads to the loss of quantum states, making it difficult to perform calculations reliably.
- What are the implications of scalability challenges? Scalability issues hinder the development of large-scale quantum computers that can perform complex tasks effectively.
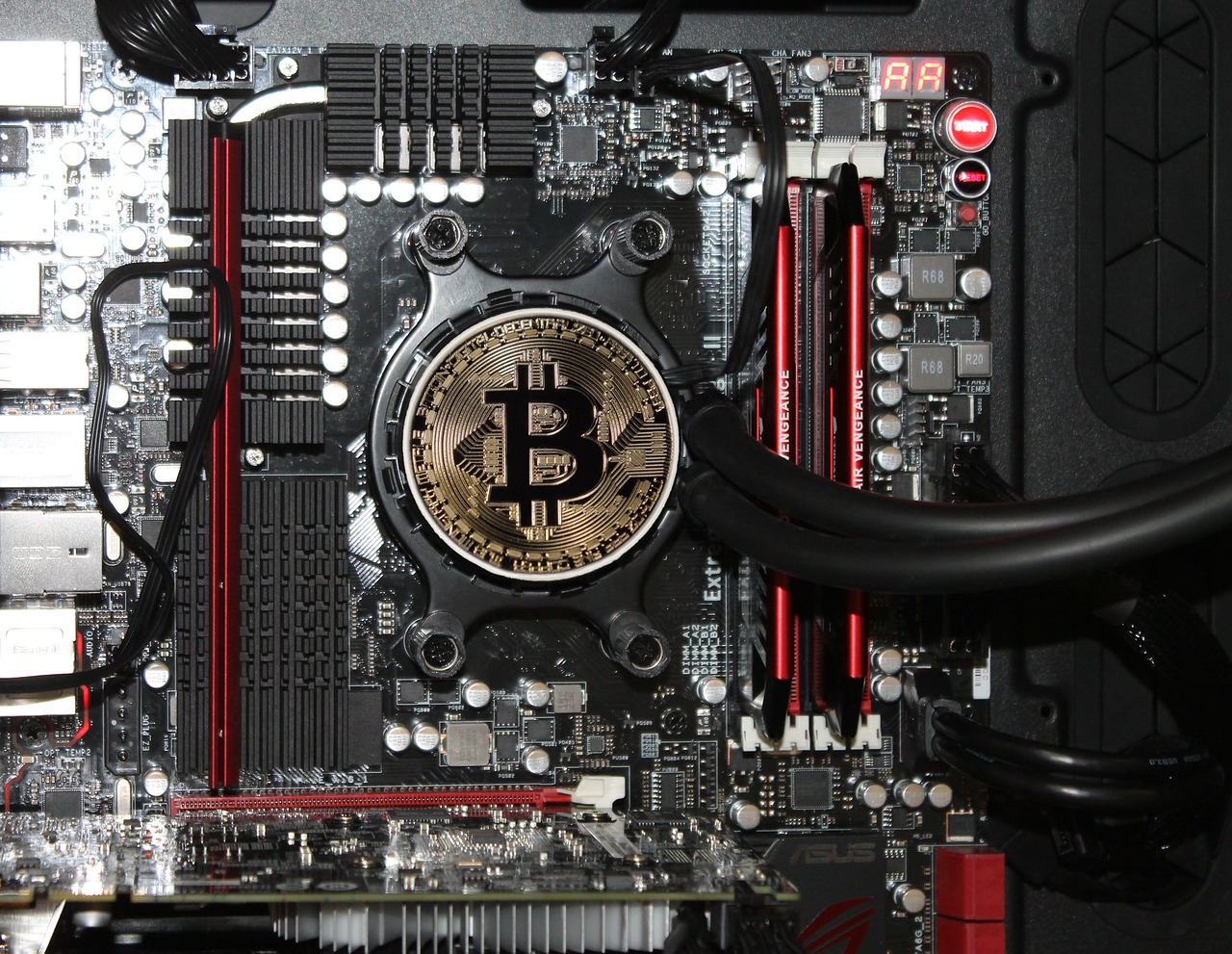
Error Correction Techniques
In the fascinating realm of quantum computing, one of the most pressing challenges is ensuring the reliability of computations. Unlike classical bits, which are either 0 or 1, qubits can exist in multiple states simultaneously, making them incredibly powerful but also susceptible to errors. This is where quantum error correction techniques come into play. They are essential for maintaining the integrity of quantum information and enabling practical applications of quantum computers.
Imagine trying to carry a glass of water while walking on a tightrope. The slightest wobble could lead to a spill, just like how environmental factors can disrupt qubits. Quantum error correction is akin to having a safety net below the tightrope, ensuring that even if something goes wrong, the qubits can be restored to their original state. This is crucial because, without effective error correction, quantum computers would struggle to perform reliable calculations over extended periods.
There are several techniques currently being explored to combat errors in quantum computing. One of the most notable methods is the Shor Code, which encodes a single logical qubit into multiple physical qubits. This redundancy allows the system to detect and correct errors without losing the original information. Another technique is the Surface Code, which uses a two-dimensional lattice of qubits to create a robust framework for error correction. This method is particularly appealing because it can be implemented with existing quantum hardware.
Here's a brief overview of some of the most prominent error correction techniques:
Technique | Description | Advantages |
---|---|---|
Shor Code | Encodes one logical qubit into nine physical qubits. | Can correct arbitrary single-qubit errors. |
Surface Code | Utilizes a 2D grid of qubits for error correction. | Scalable and highly efficient for practical implementations. |
Steane Code | Implements a seven-qubit code to protect one logical qubit. | Can correct multiple types of errors simultaneously. |
Despite the promise of these techniques, challenges remain. Error rates in quantum systems can be quite high, and as qubit numbers increase, the complexity of managing errors escalates. Researchers are actively working on developing more sophisticated algorithms and techniques to enhance the effectiveness of quantum error correction. The goal is to create a fault-tolerant quantum computer capable of performing complex calculations reliably.
In conclusion, error correction techniques are not just a safety net; they are the backbone of quantum computing's future. As we venture deeper into this revolutionary field, overcoming these challenges will be crucial for unlocking the full potential of quantum technologies. The journey is fraught with obstacles, but with each breakthrough, we move closer to a world where quantum computing can truly shine.
- What is quantum error correction? Quantum error correction involves techniques to protect quantum information from errors due to decoherence and other quantum noise.
- Why is error correction important in quantum computing? Without effective error correction, quantum computations would be unreliable, limiting the technology's practical applications.
- What are some common error correction techniques? Some common techniques include the Shor Code, Surface Code, and Steane Code, each with unique approaches to maintaining qubit integrity.
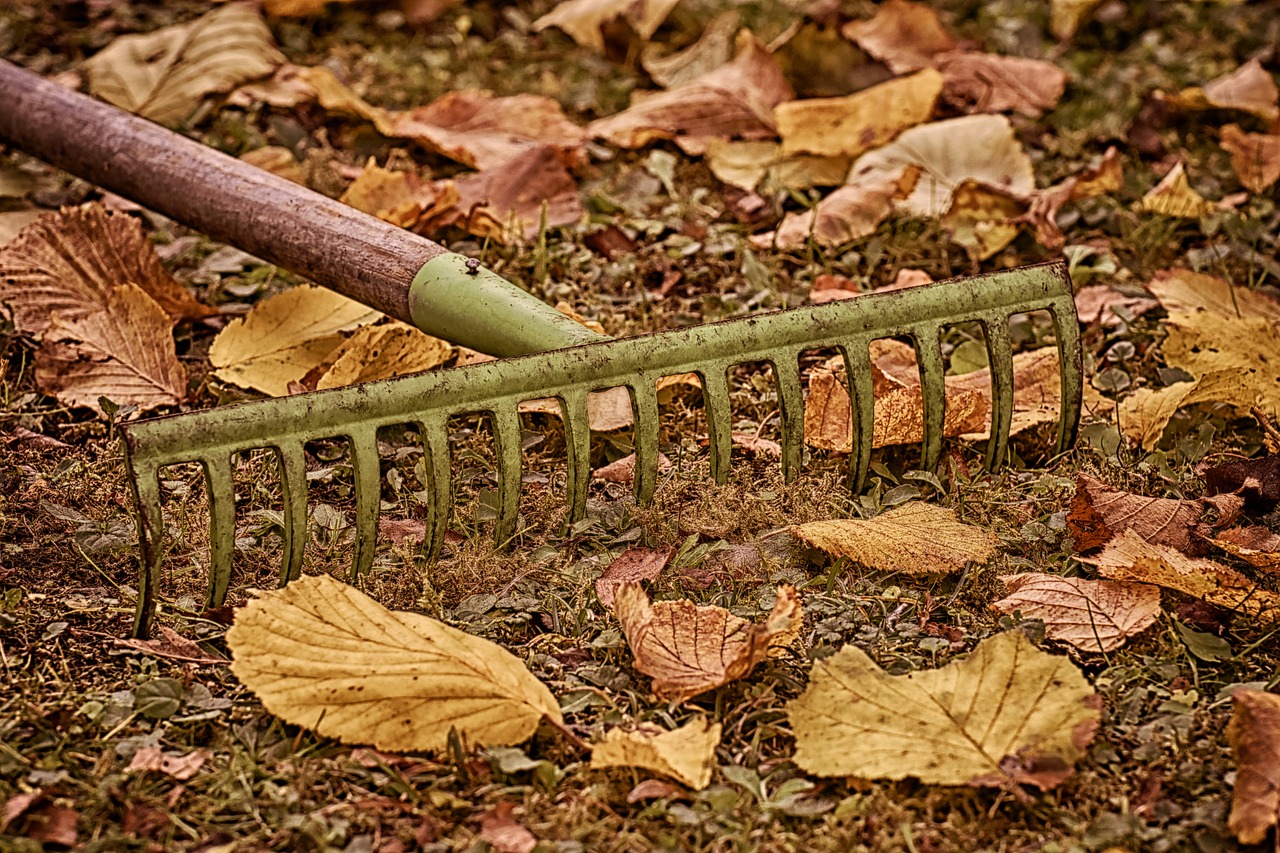
Scalability Issues
When we talk about quantum computing, we often get swept away by the dazzling potential it holds. However, like a shiny new toy, it comes with its own set of challenges that can make it difficult to play with. One of the most pressing issues is scalability. You might be wondering, why is scalability such a big deal? Well, in the world of quantum computing, it’s not just about having a few qubits here and there; it’s about how to effectively scale these systems to handle real-world problems.
To illustrate this, think of quantum computers like a budding tree. At first, it may look small and manageable, but as it grows, it requires a strong foundation and a solid structure to support its branches. Similarly, as quantum computers scale up, they face several hurdles:
- Hardware Limitations: The current physical implementations of qubits—whether they’re superconducting qubits, trapped ions, or others—have inherent limitations. These limitations can hinder the ability to increase the number of qubits without sacrificing performance.
- Quantum Interference: As more qubits are added, the complexity of interactions between them increases. This can lead to unwanted quantum interference, which can degrade the performance of the computer.
- Decoherence: Qubits are notoriously fragile. They can lose their quantum state due to interactions with their environment, a phenomenon known as decoherence. As we scale up, maintaining coherence becomes increasingly challenging.
Now, let’s take a closer look at a couple of these challenges. The hardware limitations are particularly crucial because they dictate how many qubits can be effectively managed. For instance, superconducting qubits require extremely low temperatures to function, which complicates the design of larger systems. On the other hand, trapped ions, while promising, need precise laser control, which can become unwieldy as the number of ions increases.
Decoherence poses a similar conundrum. Imagine trying to keep a room full of teenagers quiet during a party. The more people you add, the harder it becomes to maintain order. In quantum computing, the more qubits you have, the more interactions there are that can lead to decoherence, ultimately affecting the reliability of computations.
To tackle these scalability issues, researchers are exploring various strategies, including:
- Quantum Error Correction: Developing advanced error-correcting codes that can help maintain the integrity of quantum information.
- Modular Quantum Computing: Creating smaller, interconnected quantum processors that can work together to solve larger problems.
In conclusion, while the road to scalable quantum computing is fraught with challenges, it’s also filled with opportunities for innovation. The quest to overcome these scalability issues is not just about building bigger and better quantum computers; it’s about laying the groundwork for a future where quantum technology can truly shine. As researchers continue to push the boundaries, we can only imagine the revolutionary breakthroughs that lie ahead.
- What is scalability in quantum computing?
Scalability refers to the ability to increase the number of qubits in a quantum computer while maintaining performance and reliability. - What are the main challenges in scaling quantum computers?
The main challenges include hardware limitations, quantum interference, and decoherence. - How can quantum error correction help?
Quantum error correction techniques are designed to protect quantum information from errors, thereby improving the reliability of quantum computations.
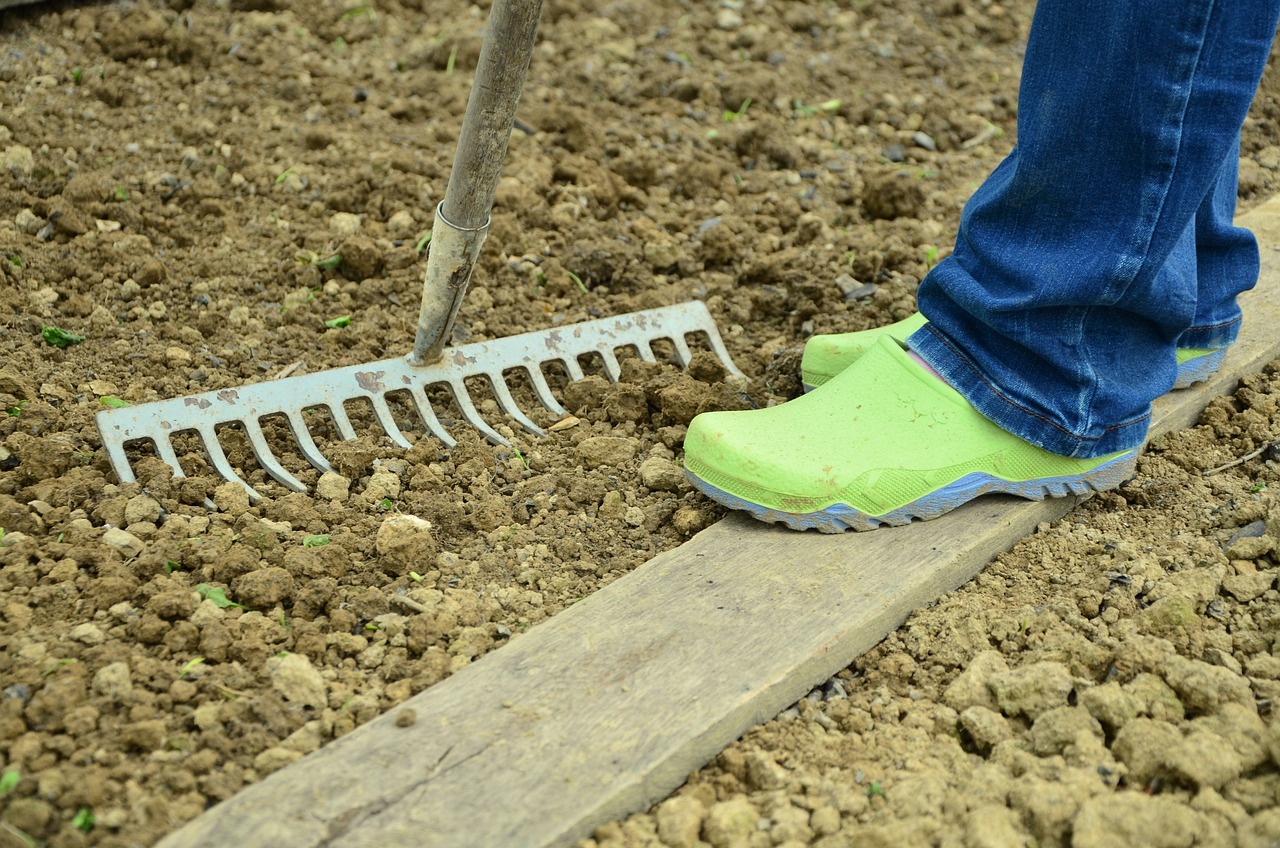
The Future of Quantum Computing
The future of quantum computing is not just a realm of speculation; it is a burgeoning landscape filled with possibilities that could redefine our technological boundaries. Imagine a world where complex problems, which currently take classical computers thousands of years to solve, are handled in mere seconds. This isn't science fiction—it's the potential reality that quantum computing brings to the table. As researchers and companies dive deeper into this fascinating field, we can expect significant breakthroughs that will not only change the way we compute but also how we approach problems across various industries.
One of the most exciting prospects is the potential for quantum supremacy. This term refers to the point at which quantum computers can solve problems that classical computers practically cannot, regardless of the time they are given. Major tech companies, including Google and IBM, are racing to achieve this milestone, and their efforts will likely yield innovations that ripple across sectors such as finance, healthcare, and logistics. For instance, in drug discovery, quantum computing could simulate molecular interactions at an unprecedented scale, leading to faster and more efficient development of new medications.
Moreover, as quantum technologies mature, we can expect a wave of commercialization. Companies are already investing heavily in quantum research, with many setting up dedicated teams to explore practical applications. This influx of investment will likely lead to the development of quantum-as-a-service platforms, where businesses can access quantum computing power via the cloud. Such accessibility will democratize the technology, allowing smaller firms to leverage quantum capabilities without the need for extensive infrastructure.
However, as we look towards a quantum-enabled future, we must also consider the ethical implications of these advancements. The ability to break traditional encryption methods poses significant risks to data security. As quantum computers become more powerful, the urgency for developing quantum-resistant encryption methods grows. This creates a dual challenge: not only must we advance quantum technology, but we must also safeguard against its potential misuse.
In addition to ethical concerns, there are practical considerations regarding industry adoption. Various sectors are gearing up for the quantum revolution, each with unique challenges and opportunities:
Industry | Potential Applications | Challenges |
---|---|---|
Healthcare | Drug discovery, genomics | Data privacy, integration with existing systems |
Finance | Risk analysis, portfolio optimization | Scalability, regulatory compliance |
Logistics | Supply chain optimization, route planning | Infrastructure readiness, cost |
As we navigate these complexities, it's essential to foster collaboration between technologists, ethicists, and policymakers. Only through a united approach can we ensure that quantum computing serves as a force for good, driving innovation while safeguarding societal values.
In conclusion, the future of quantum computing is bright, filled with both challenges and opportunities. As we stand on the brink of this technological revolution, it’s crucial to remain informed and engaged with the developments in this field. The next few years will likely see quantum computing transition from theory to practice, with profound implications for how we live and work.
- What is quantum computing? Quantum computing is a type of computation that utilizes quantum bits (qubits) to perform calculations at speeds unimaginable with classical computers.
- When will quantum computers be widely available? While significant progress is being made, widespread availability may still be several years away, depending on advancements in technology and infrastructure.
- How will quantum computing affect data security? Quantum computing has the potential to break current encryption methods, prompting the need for new, quantum-resistant security protocols.
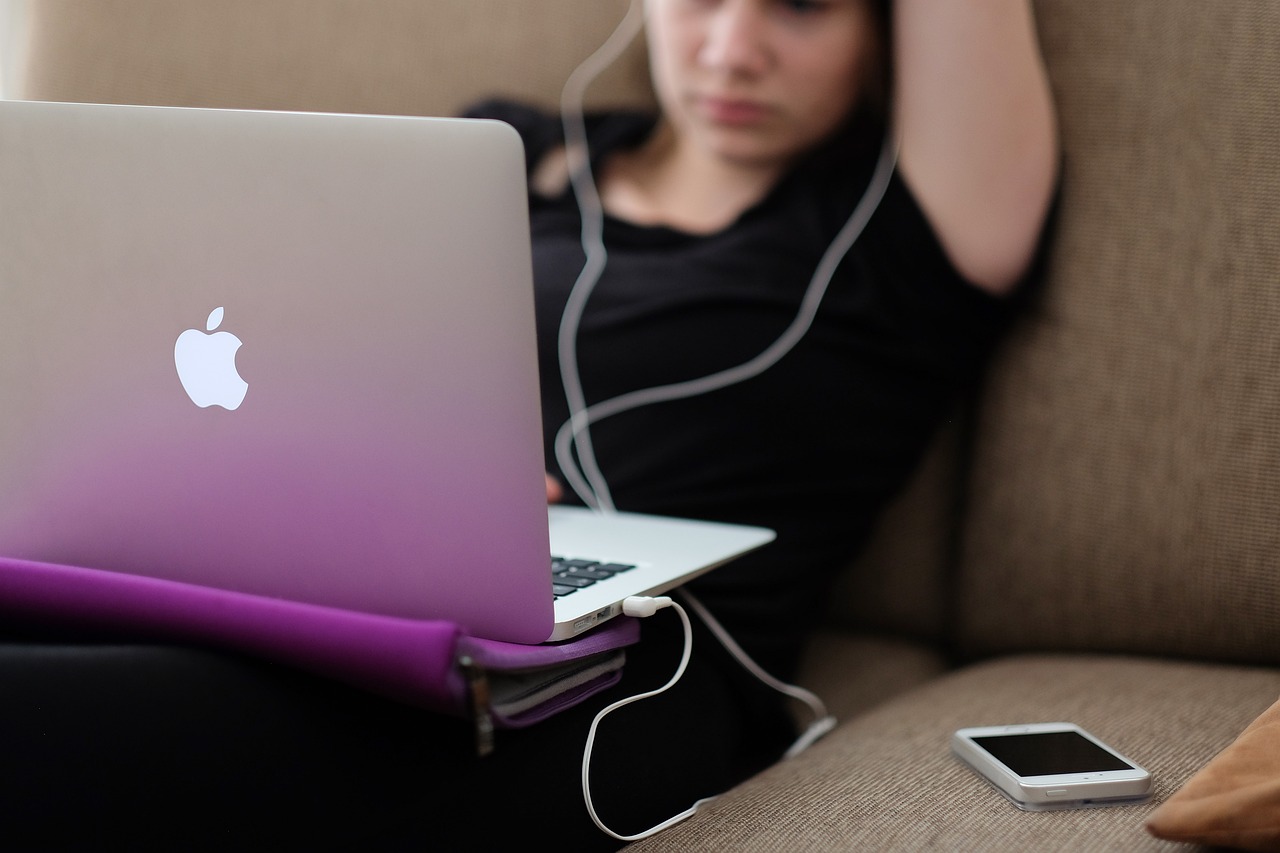
Industry Adoption
As we stand on the brink of a technological revolution, quantum computing is not just a theoretical concept confined to the walls of research labs; it's making its way into various industries at an astonishing pace. Companies across sectors are beginning to recognize the immense potential of quantum technologies to solve complex problems that were previously deemed unsolvable. Think of it as a new toolkit that can tackle challenges ranging from cryptography to drug discovery, all while operating at speeds that classical computers can only dream of.
One of the most significant adopters of quantum computing technology is the financial sector. Banks and financial institutions are exploring quantum algorithms to optimize trading strategies, manage risk, and even detect fraud. For instance, JPMorgan Chase has been actively investing in quantum research, aiming to leverage this technology to gain a competitive edge in the market. Imagine being able to analyze vast amounts of market data in seconds—this is the kind of transformation that quantum computing promises.
In the realm of pharmaceuticals, companies like Pfizer and Roche are utilizing quantum computing to enhance drug discovery processes. By simulating molecular interactions at unprecedented speeds, these companies can significantly reduce the time it takes to bring new drugs to market. This is akin to having a supercharged laboratory assistant that can run countless experiments simultaneously, leading to faster breakthroughs in medicine.
Moreover, the logistics and supply chain management sectors are also beginning to embrace quantum technologies. Companies such as DHL and IBM are experimenting with quantum algorithms to optimize delivery routes and inventory management. The ability to compute the most efficient routes in real-time could revolutionize how goods are transported, leading to reduced costs and improved customer satisfaction. It's like having a GPS that not only knows where you are but can also predict traffic patterns and optimize your journey before you even start.
However, the journey toward widespread adoption is not without its hurdles. Companies must navigate the complexities of integrating quantum computing into their existing infrastructures. This often requires significant investment in both technology and talent. The current shortage of skilled quantum programmers and researchers poses a significant challenge, as organizations scramble to find experts who can translate quantum theory into practical applications.
To illustrate the current landscape of industry adoption, we can look at the following table showcasing various sectors and their respective quantum initiatives:
Industry | Company | Quantum Initiative |
---|---|---|
Finance | JPMorgan Chase | Quantum algorithms for risk analysis |
Pharmaceuticals | Pfizer | Accelerating drug discovery |
Logistics | DHL | Optimizing delivery routes |
Telecommunications | AT&T | Enhancing network security |
Energy | BP | Improving energy grid management |
In conclusion, while the adoption of quantum computing across industries is still in its infancy, the momentum is undeniable. As more companies recognize the transformative power of this technology, we can expect to see a surge in innovation and efficiency. The future is bright, and as quantum computing continues to evolve, its impact on industries and society will be profound.
Q: What is quantum computing?
A: Quantum computing is a type of computing that uses quantum bits (qubits) to perform calculations at incredible speeds, leveraging the principles of quantum mechanics.
Q: How is quantum computing different from classical computing?
A: Unlike classical computers that use bits as the smallest unit of information (0 or 1), quantum computers use qubits, which can exist in multiple states simultaneously due to superposition.
Q: What industries are currently adopting quantum computing?
A: Industries such as finance, pharmaceuticals, logistics, telecommunications, and energy are actively exploring and integrating quantum computing technologies into their operations.
Q: What are some challenges in adopting quantum computing?
A: Major challenges include the need for skilled professionals, the complexity of integrating quantum systems with existing infrastructures, and addressing issues related to error rates and scalability.
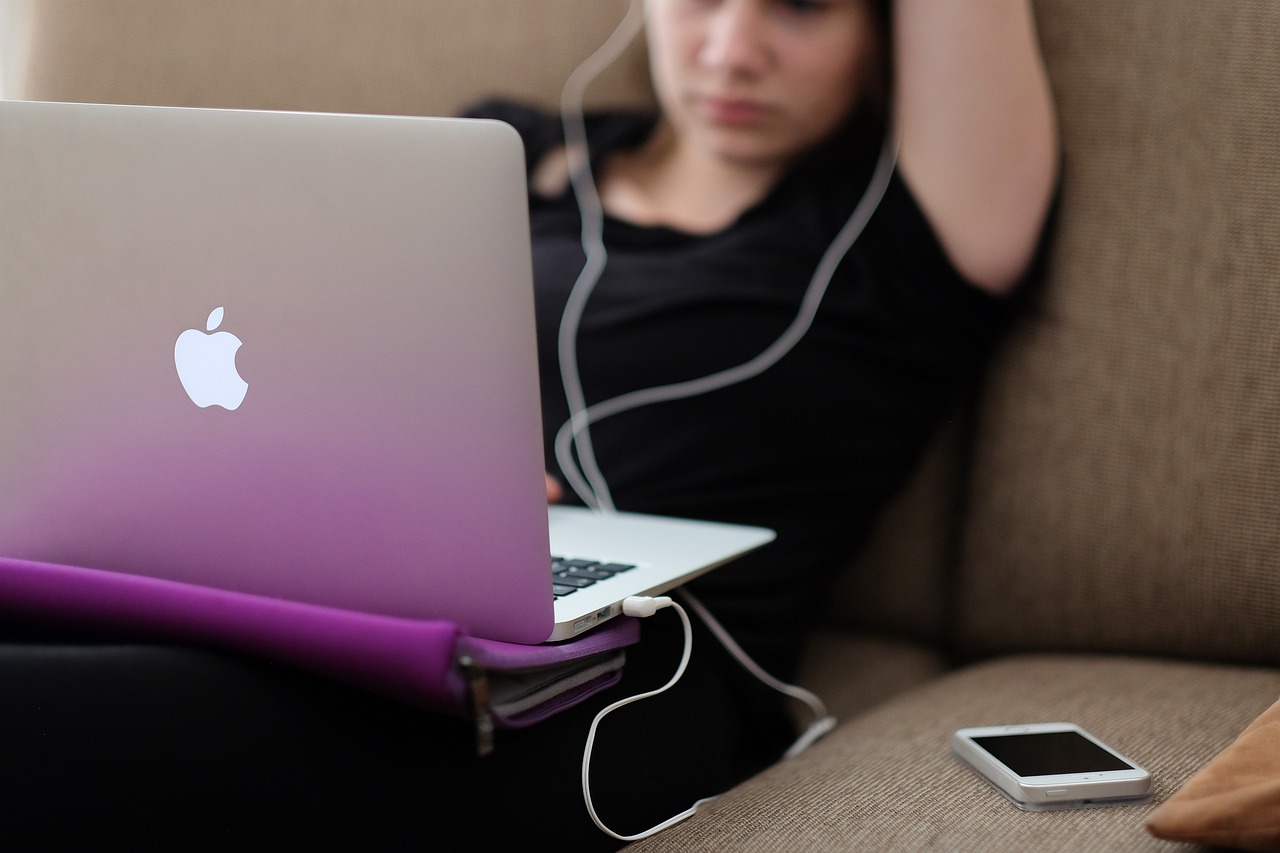
Societal Implications
As we stand on the brink of a technological revolution, quantum computing promises to transform not only how we process information but also the very fabric of our society. Imagine a world where complex problems that currently take years to solve can be tackled in mere seconds! This potential brings with it a host of societal implications that we must consider carefully.
First and foremost, the rise of quantum computing will significantly impact industries across the board. From finance to healthcare, the ability to analyze vast datasets and optimize processes will lead to unprecedented efficiency. For instance, in drug discovery, quantum algorithms could simulate molecular interactions at a level of detail that classical computers simply cannot achieve. This means faster development of life-saving medications, ultimately leading to improved public health outcomes.
However, with great power comes great responsibility. The implications for privacy and security are profound. Quantum computers have the potential to break current encryption methods, putting sensitive data at risk. As organizations scramble to adopt quantum-resistant encryption techniques, there will be a significant shift in how we approach data security. This transition could create a new landscape of cyber threats, where both individuals and corporations must remain vigilant.
Moreover, the rapid development of quantum technologies raises ethical questions. As we integrate quantum computing into various sectors, we must consider the digital divide. Will access to this powerful technology be equitable? Or will it exacerbate existing inequalities? Governments and organizations must strive to ensure that advancements in quantum computing benefit all, not just a select few. This might involve investing in education and training programs to prepare a workforce capable of navigating this new landscape.
Additionally, the potential for job displacement cannot be ignored. As quantum computing automates complex tasks, some jobs may become obsolete. However, this also opens up new opportunities in fields that did not exist before. For example, roles focused on quantum algorithm development, quantum hardware engineering, and even quantum ethics will emerge. Society must be proactive in retraining workers and creating pathways to new careers in this evolving job market.
Finally, the societal implications of quantum computing extend to our global landscape. As countries race to achieve quantum supremacy, geopolitical dynamics may shift. Nations that lead in quantum technology could gain significant advantages in defense, intelligence, and economic power. This competition could lead to a new arms race, where technological prowess is as important as military might.
In conclusion, while the promise of quantum computing is exhilarating, it is crucial to navigate its societal implications thoughtfully. By fostering equitable access, addressing ethical concerns, and preparing the workforce for the future, we can harness the power of quantum computing for the greater good. The journey ahead is filled with challenges, but with careful consideration and proactive measures, we can ensure that this revolutionary technology benefits all of humanity.
- What is quantum computing? Quantum computing is a field of computing that utilizes the principles of quantum mechanics to process information in fundamentally different ways compared to classical computers.
- How will quantum computing affect cybersecurity? Quantum computers can potentially break traditional encryption methods, necessitating the development of quantum-resistant security measures to protect sensitive data.
- Will quantum computing create job opportunities? Yes, while some jobs may be displaced, quantum computing will also create new roles in algorithm development, hardware engineering, and more.
- What are the ethical implications of quantum computing? The ethical implications include issues of access, privacy, and the potential for increased inequality if the technology is not distributed equitably.
Frequently Asked Questions
- What is quantum computing?
Quantum computing is a groundbreaking technology that leverages the principles of quantum mechanics to process information. Unlike classical computers, which use bits as the smallest unit of data, quantum computers use qubits. This allows them to perform complex calculations at speeds unimaginable with conventional technology.
- How do qubits differ from classical bits?
Classical bits can exist in one of two states, 0 or 1, whereas qubits can exist in a state of superposition, meaning they can be both 0 and 1 simultaneously. This unique property enables quantum computers to explore multiple solutions at once, drastically increasing their computational power.
- What are some practical applications of quantum computing?
Quantum computing has the potential to revolutionize various fields, including cryptography, where it can break traditional encryption methods, optimization problems in logistics and finance, and drug discovery, allowing researchers to simulate molecular interactions quickly.
- What are the main challenges facing quantum computing?
Despite its promise, quantum computing faces significant challenges, including error rates due to decoherence, the difficulty of maintaining qubit stability, and the need for advanced quantum error correction techniques to ensure reliable computations.
- How does quantum error correction work?
Quantum error correction involves encoding qubits in such a way that errors can be detected and corrected without measuring the qubits directly. This is crucial for maintaining the integrity of quantum computations over time, as it helps counteract the effects of decoherence and other noise.
- What types of qubits are there?
There are several types of qubits, including superconducting qubits, which are highly efficient and widely used, trapped ions, which offer excellent coherence times, and topological qubits, which aim to provide greater stability against errors. Each type has its own advantages and challenges.
- What is the future of quantum computing?
The future of quantum computing is promising, with ongoing research aimed at overcoming current limitations. As technology advances, we can expect increased industry adoption, leading to transformative impacts on sectors like finance, healthcare, and logistics, as well as broader societal implications.
- How is the industry preparing for quantum computing?
Many industries are investing in research and development to integrate quantum computing into their operations. This includes forming partnerships with tech companies, funding academic research, and training personnel to ensure they can leverage this technology effectively as it matures.
- What are the ethical considerations of quantum computing?
Quantum computing raises several ethical questions, particularly regarding privacy and security. As it can potentially break current encryption methods, there is a pressing need to develop new standards and practices to protect sensitive information in a quantum-enabled world.